- Department of Chemistry and Biochemistry
- Research
- Faculty Interests
Faculty Research Interests
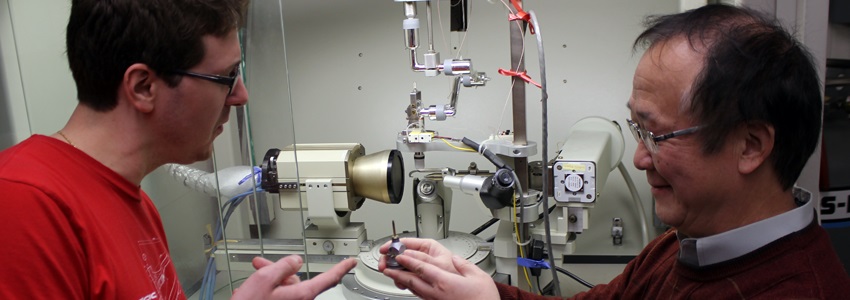
Students in our department experience a wide range of research opportunities — from working with a single faculty advisor in the traditional areas of chemistry, through highly interdisciplinary and collaborative projects.
The research interests of our faculty not only span across the traditional areas of chemistry, but also across other disciplines, including materials science, biology, physics and engineering.
![]() |
Elizabeth R. GaillardPh.D., University of Texas at Austin, 1991 Light Damage in Biological TissuesOur research interests lie in the general area of the biochemical basis of age related eye diseases such as cataract and macular degeneration. We are particularly interested in understanding the rates and mechanisms of chemical reactions that ultimately lead to tissue damage, because this information allows us to develop drugs or protocols that can slow down, stop or even possibly reverse injury. The eye is continuously exposed to light, has the most (retina) and least (lens) oxygenated tissue in the body and has highly specialized structures for tight regulation of material into and out of the eye. These factors all play important roles in the onset and progression of disease. Our work involves the use of several types of laser based spectroscopy, mass spectrometry (lipidomics and proteomics) and tissue culture methods. |
![]() |
Thomas M. GilbertPh.D., University of California, Berkeley, 1985 Computational ChemistryResearch in the Gilbert group focuses on computationally elucidating why compounds react as they do. We employ electronic structure theory to address questions involving a range of donor/acceptor interactions, including trans‐annular interactions in cage compounds and weak interactions in “frustrated Lewis pair” systems. We also study compounds that formally contain donor/acceptor multiple bonds, comparing their structures and reactivities to those of well‐known species such as alkenes/alkynes and transition metal carbenes/carbynes. We collaborate in diverse areas including organic, inorganic, and analytical chemistries by supporting experimental work with computational studies, providing physical underpinnings to laboratory observations. |
![]() |
Timothy J. HagenPh.D., University of Wisconsin—Milwaukee, 1988 Design and Synthesis of Molecules with Biological SignificanceOur lab is focused on structure‐based design and synthesis of small molecules that can modulate essential pathways of infectious disease organisms with an emphasis on small molecule–protein interactions and target specificity. We utilize fragmentbased screening, de novo design and synthesis of natural products. Specific areas of interest include: fragment‐based design of novel small molecules to selectively disrupt key enzymatic interactions such as the non‐mevalonate isoprenoid biosynthetic pathway; design and synthesis of novel small‐molecules to selectively inhibit enzymes such as MetAP2 that are essential to bacteria and parasitic organisms; and natural product synthesis with investigation of their biological activities. |
![]() |
Oliver HofstetterDr. rer. nat., Eberhard‐Karls‐Universität Tübingen, 1999 Biorecognition and Chiral SeparationsPhysiological processes such as metabolic pathways, intra‐ and intercellular communication, and the response to infection depend on highly specific interactions between biological macromolecules and their binding partners, called ligands. Research in the Hofstetter lab focuses on the exploitation of the unsurpassed binding characteristics of proteins in bioanalytical applications ranging from affinity chromatography to fingermark detection. Both classical immunological and molecular biological techniques are employed to produce affinity capture reagents and optimize their physicochemical properties. An important aspect of our work is the design of novel assays, biosensors and chromatographic techniques that then utilize these reagents for the detection and separation, respectively, of relevant ligands. |
![]() |
James R. HornPh.D., University of Iowa, 2002 Protein Interactions and Protein EngineeringProtein–protein and protein–ligand interactions play fundamental roles in biological processes and are frequently used in a variety of biotechnology applications. Consequently, there is great interest in understanding the chemical properties which drive these interactions and learning how they may be manipulated. Using a combination of experimental, computational and protein engineering methods, our lab addresses these topics by examining the role of protein structure, dynamics and stability on the binding energetics. We are also interested in developing new methodologies that allow “customized” control of protein interactions. The long‐term impact of this research will help enhance our basic understanding of bimolecular recognition, improve protein engineering and design efforts, in addition to aiding structure‐based drug design. |
![]() |
Douglas A. KlumppPh.D., Iowa State University, 1993 Synthetic Organic ChemistryOur research is multifaceted. While the work is centered in organic chemistry, we have interests in all aspects of this field. The research involves both synthetic methodology development (inventing new chemical reactions) and target‐driven organic synthesis (natural products and pharmaceutical intermediates). Recent work in our laboratory has also sought to develop synthetic methods in bio‐renewable chemistry. We also conduct many studies related to organic reaction mechanisms, with a strong emphasis in the study of electrophile–nucleophile reactions and acidcatalyzed processes. These studies utilize many of the tools available in physical organic chemistry, including theoretical calculations, spectroscopic studies, isotopic labeling and kinetic studies. By being involved in mechanistic studies, our research group is able to use this knowledge to inspire new synthetic chemistry. |
![]() |
Tao LiPh.D., University of South Carolina–Columbia, 2009 Bio‐inspired Functional Nanomaterials for Medical and Energy ApplicationsOur research encompasses interdisciplinary projects to design and synthesize novel hierarchical functional nanomaterials for potential applications in nanomedicine and energy related fields. We have a broad interest in nanocatalyst, electrolyte, nanoparticle synthesis and assembly, drug/protein delivery, enzyme immobilization and synchrotron characterization. Our group will have opportunities to interact with the leading scientists at Argonne and learn advanced characterization tools at APS, CNM and EMC. |
![]() |
Evgueni E. NesterovPh.D., Moscow State University, 1996 Functional Organic Materials and PolymersResearch in our group encompasses various areas of experimental and theoretical organic chemistry, functional organic materials and polymers, physical organic chemistry and photochemistry. Among specific directions are: (1) Development of new methods to synthesize semiconducting polymers and polymer nanostructures. (2) Design of fluorescent sensors. (3) Application of neutron and X-ray scattering based methods towards studies of structure and properties of semiconducting polymer materials. (4) Energy transfer in conjugated polymers and small molecules – theory and practical applications. (5) Nanostructured thin films and organic optoelectronic devices. (6) Molecular photochemistry – novel photochemical reactions, photochemistry in organized and constrained media.
|
![]() |
Irina NesterovaPh.D., Moscow State University, 1997 Bioanalytical Sensing SystemsResearch in our group evolves around development of new sensing systems for biological applications. Mostly we focus on designing molecular devices based on DNA backbone. DNA structure is refined over billions of years to be biocompatible, stable, and programmable material capable to interact with different ligands. For example, we are building upon DNA i‐motif';s ability to bind protons to develop sensing devices capable to detect very small changes in concentration of H+ in vivo as well as in vitro for indirect assessment of processes involving protons (e.g. DNA replication, RNA transcription, phosphorylation). In addition, we work on the development of sensing systems that combine functionality of small molecules with advantages characteristic for oligonucleotides. We utilize variety of spectroscopic (fluorescence, UV‐vis absorption) and separation techniques (electrophoresis, chromatography), microscopy and other methods to study and characterize the sensing systems. |
![]() |
Victor RyzhovPh.D., Case Western Reserve University, 1998 Bioanalytical Mass SpectrometryResearch in the Ryzhov group focuses on bioanalytical applications of mass spectrometry and gas‐phase ion chemistry. One area of interest involves studying structure and reactivity of radical ions in systems of biological interest (amino acids, peptides, nucleotides). This highly collaborative project uses ion–molecule reactions, computational chemistry and ion IR spectroscopy to assess fundamental processes related to free radicals in proteins and DNA. Another application deals with gas‐phase catalysis using metal ion complexes. Examples of this research include developing new catalysts for C–C bond coupling and hydrogen storage via the formic acid cycle. Our group also collaborates with Prof. Hagen on developing HPLC and LC/MS assays for monitoring enzyme inhibition. |
![]() |
Lee S. SunderlinPh.D., University of California, Berkeley, 1990 Mass Spectrometry and ThermodynamicsMass spectrometry can be used to make precise measurements of physical properties of molecules. The flowing afterglow‐guided ion beam mass spectrometer in our laboratory is used to study the reactions of ions with neutrals at well‐defined energies. One main experiment is energy‐resolved collision‐induced dissociation, where the energy required for bond dissociation is measured. Systems studied range from species of atmospheric importance such as sulfuric acid to examples of hypervalent bonding such as IF8 and are being extended to larger species of biochemical interest. Computational chemistry can also be applied to these systems. One of the main results of these experiments is the determination of periodic trends, taking advantage of the ability of the mass spectrometer to handle periodically related ions by simply switching masses. |
![]() |
Ralph A. WheelerPh.D., Cornell University, 1988 Modeling Molecular Energy Storage, Transduction and UseEnergy storage, change, and its eventual use is vital to living organisms and to our progress as a society. My research group';s interests have evolved from simulating electron transfer proteins to include modeling and optimizing (1) the mechanisms of metalloenzyme reactions (e.g. enzymatic conversion of methane greenhouse gas to methanol chemical feedstock) and (2) the properties of electrolytes (including ionic liquids) for battery applications. Students in my group typically learn quantum chemical and molecular dynamics simulations. We also develop new ways to analyze and visualize simulation results. Our work is interdisciplinary and accommodates a range of interests in chemistry/ biochemistry, computers, physics, statistics, and/or signal processing. |
![]() |
Tao XuPh.D., University of Alabama, 2003 Hybrid Interfaces and Nanomaterials for Clean Energy ApplicationsResearch in Dr. Xu';s group pursues a fundamental understanding in photoelectrochemistry and material science related to solar photovoltaic energy conversion, photocatalytic oxidation for environmental purification, and nanocatalysts for energy storage, utilization and green chemical production. These systems involve intricate interfacial nanostructures between various materials, which exhibit profound influence on their performances. Our research focus is to investigate the basic science associated with the nanoscale charge transfers and migration of atoms at various hybrid interfaces. This provides a solid foundation to strategically build our design, syntheses and fabrication of materials and devices that are capable of facilitating the desired transfers while suppressing the unwanted transfers for enhanced performance of the above systems. |
Contact Us
Northern Illinois University
Department of Chemistry and Biochemistry
Faraday Hall, Room 319
DeKalb, IL 60115